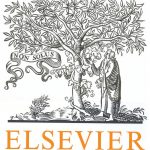
ترجمه مقاله نقش ضروری ارتباطات 6G با چشم انداز صنعت 4.0
- مبلغ: ۸۶,۰۰۰ تومان
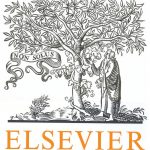
ترجمه مقاله پایداری توسعه شهری، تعدیل ساختار صنعتی و کارایی کاربری زمین
- مبلغ: ۹۱,۰۰۰ تومان
ABSTRACT
Bone resorption by osteoclasts occurs through the creation of a sealed extracellular compartment (ECC), or pit, adjacent to the bone that is subsequently acidified through a complex biological process. The low pH of the pit dissolves the bone mineral and activates acid proteases that further break down the bone matrix. There are many ion channels, transporters, and soluble proteins involved in osteoclast mediated resorption, and in the past few years, there has been an increased understanding of the identity and properties of some key proteins such as the ClC-7 Cl−/H+ antiporter and the HV1 proton channel. Here we present a detailed mathematical model of osteoclast acidification that includes the influence of many of the key regulatory proteins. The primary enzyme responsible for acidification is the vacuolar H+-ATPase (V-ATPase), which pumps protons from the cytoplasm into the pit. Unlike the acidification of small lysosomes, the pit is so large that protons become depleted from the cytoplasm. Hence, proton buffering and production in the cytoplasm by carbonic anhydrase II (CAII) is potentially important for proper acidification. We employ an ordinary differential equations (ODE)-based model that accounts for the changes in ionic species in the cytoplasm and the resorptive pit. Additionally, our model tracks ionic flow between the cytoplasm and the extracellular solution surrounding the cell. Whenever possible, the properties of individual channels and transporters are calibrated based on electrophysiological measurements, and physical properties of the cell, such as buffering capacity, surface areas, and volumes, are estimated based on available data. Our model reproduces many of the experimental findings regarding the role of key proteins in the acidification process, and it allows us to estimate, among other things, number of active pumps, protons moved, and the influence of particular mutations implicated in disease.
5. Conclusion
We have assembled a comprehensive list of biophysical parameters from the literature that describe the conditions related to actively resorbing osteoclasts including the extracellular environment, cytoplasm, and extracellular compartment including relevant ion concentrations, geometric factors, buffering powers, and reaction rates (see Tables 1 and 2). These values are needed to construct a quantitative model of resorption, and they form a basis from which future modifications to the model can be made. The model does a good job at semi-quantitatively describing experiments involving pH changes in the cytoplasm and the extracellular compartment, and the model makes important hypotheses regarding variables that cannot be easily measured in experiment such as pump and channel numbers, membrane potential, and ionic concentration changes in time. Thus, our model has sufficient chemical detail to predict how changes in experimental setup, such as external ion concentrations, genetic mutations, and knockouts, will in- fluence experimental results; and therefore, it can be used to drive new experiments. That said, we acknowledge that there are many elements of the model that are poorly characterized, which can limit the reliability of the results. For instance, cell geometry is difficult to estimate, and we have assumed a few constant values for many of the calculations presented here. If volume or surface areas are different from what we have assumed, the estimated pump numbers will be directly affected. Additionally, the pump numbers reported here directly depend on the single protein turnover rates shown in Fig. 2. If these rates are over or underestimated, the reported copy numbers will change. Finally, we have provided our source code for researchers to freely download and modify so that they can tailor components to their experimental system and use the solutions as a tool to help interpret their results (bitbucket.org/grabe-lab/). Currently, the code is in Matlab™, and requires access to this software to run; however, in the near future, a free version in python or Julia will be provided as well.